Shunt reactors are specialized devices utilized in electrical power systems to manage reactive power, particularly in high voltage transmission lines and underground cable networks.
As power systems expand, issues such as excessive voltage arise, leading to the need for compensation mechanisms. Shunt reactors are introduced to address these issues by counteracting the leading reactive power, ensuring system stability and efficiency.
This article provides an in-depth exploration of shunt reactors, their types, operations, and the technology behind them.
The Need for Shunt Reactor
The expansion of very high voltage (VHV) transmission systems and underground cable networks leads to unwanted reactive power, specifically leading reactive power. This can cause over-voltage situations, especially during low load conditions often called as Ferranti effect.
Shunt reactors, primarily switched in during periods of low demand, provide the necessary compensation by absorbing the excess reactive power. The main function of shunt reactor is reactive power compensation.
Series reactors on the other hand are used to limit short-circuit currents and manage power flow.
Shunt Reactor Connection
The image below demonstrates how shunt reactors are integrated into the power system to enhance voltage stability and protect against transients, contributing to the overall reliability of the electrical network.
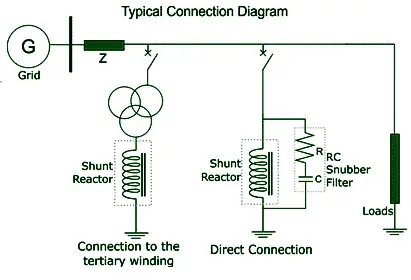
Types of Connections:
- Direct Connection to Transmission Line:
- Shunt reactors can be directly connected in parallel with the transmission line.
- This allows them to absorb reactive power directly from the line, stabilizing voltage levels.
- Connection to Tertiary Winding of Transformer:
- Alternatively, shunt reactors can be connected to the tertiary winding of a transformer.
- This configuration allows for reactive power absorption from the transformer, helping to manage voltage levels without direct interference with the main transmission line.
- RC Snubber Filter:
- Often used in conjunction with directly connected shunt reactors.
- The filter helps to protect the power system by damping transients and reducing oscillations.
- By absorbing excess reactive power, shunt reactors prevent overvoltage conditions in the power system.
- They ensure stable and efficient operation of the power network, particularly during low load conditions or over long transmission lines.
Types of Shunt Reactors
Shunt reactors are generally classified into two principal types based on their core structure and design:
1. Air Core Shunt Reactors
Air core, or coreless reactors, do not have a magnetic core. Instead, the magnetic flux generated circulates within the winding itself and passes through air or another medium of unit permeability, like mineral oil.
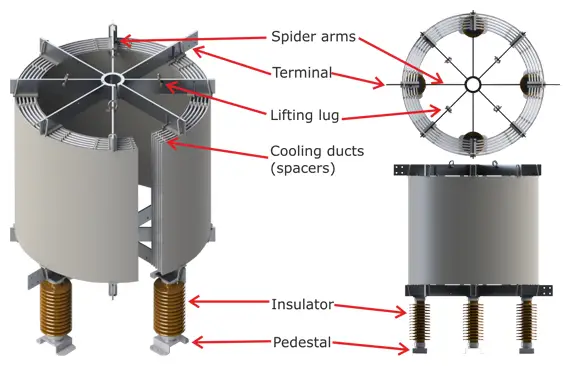
This design is typically used at lower voltages due to its simplicity and effectiveness in environments with fewer external magnetic constraints.
2. Gapped Core Shunt Reactors
Gapped core shunt reactors, on the other hand, feature a magnetic core inside and concentric with the winding.
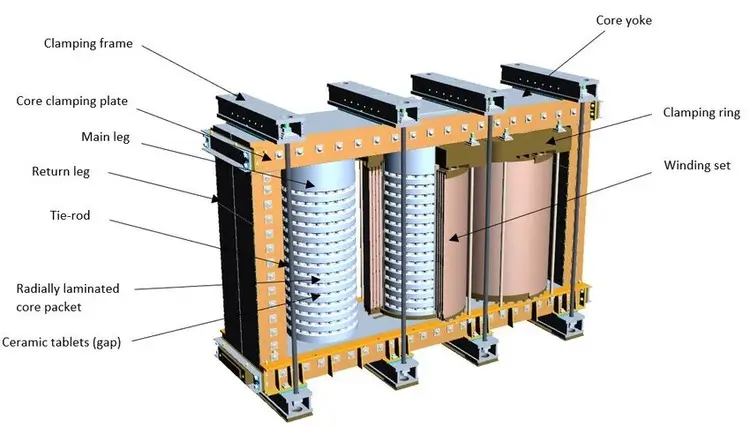
This core is constructed from radially disposed laminated steel discs, separated by gaps filled with an insulating fluid. These gaps help maintain the desired voltage-current characteristic, making this design suitable for higher voltage applications.
Working Principles of Shunt Reactors
The functionality of shunt reactors relies heavily on their core design and the magnetic properties of their components.
1. Air Core Reactors
In air core reactors, the magnetic flux circulates within the winding and through a medium of low permeability.
These reactors feature a linear magnetization characteristic, meaning their reactance remains relatively constant until the magnetic shield saturates. This saturation point usually lies well above the reactor’s rated voltage, ensuring stable performance across a wide range of operational conditions.
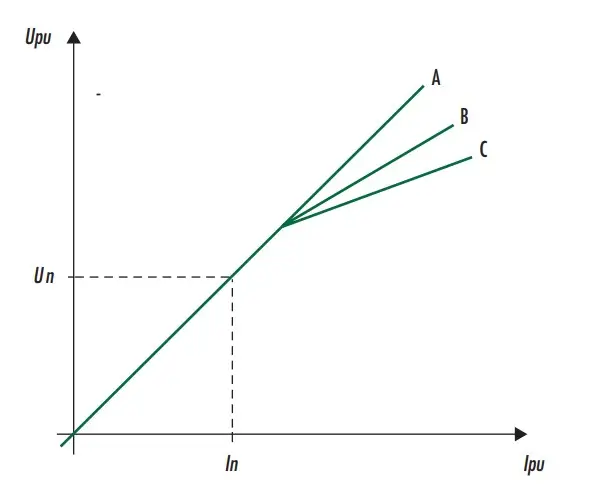
A – Theoretical (Constant Impedance)
B – Air core reactor with shield saturation at knee point voltage
C – Gapped core reactor
The above characteristic is important for the operation of the reactor during
voltage transition
2. Gapped Core Reactors
The windings in gapped core reactors are generally of disc or layer type, featuring small conductors that minimize eddy current losses and local hot spots.
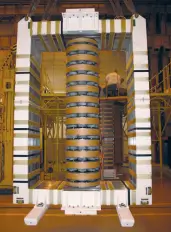
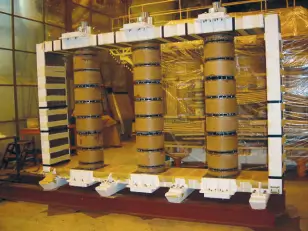
The magnetic core consists of silicon steel discs arranged radially, with the air gaps forming a critical part of the reactor’s impedance control mechanism.
Air Core Reactor Design and Characteristics
Air core reactors, often used in lower voltage applications, have a straightforward design. The cylindrical winding is typically housed within an external magnetic shield, which is designed to channel the return flux and prevent excessive heating in nearby metallic structures. The external shield can vary in shape, as long as it effectively contains the magnetic flux.
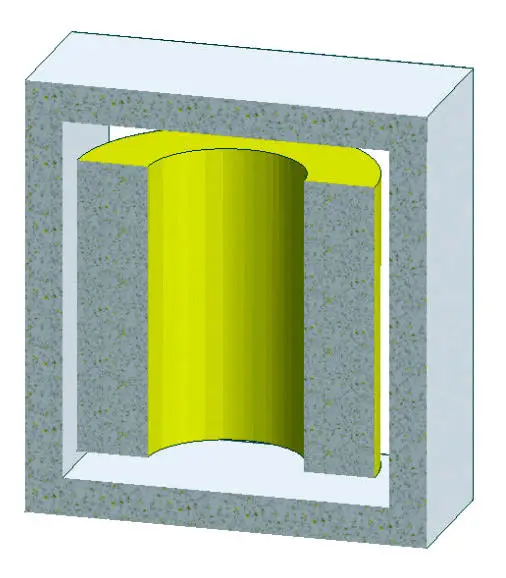
Gapped Core Reactor Design and Characteristics
Magnetic Core Structure
The core of a gapped core reactor is made up of silicon steel discs, each encapsulated in epoxy resin to reduce vibrations and noise. The discs are separated by ceramic or slate spacers, which form the critical air gaps. These air gaps play a vital role in stabilizing the magnetic flux within the reactor and maintaining a consistent impedance across different operating conditions.
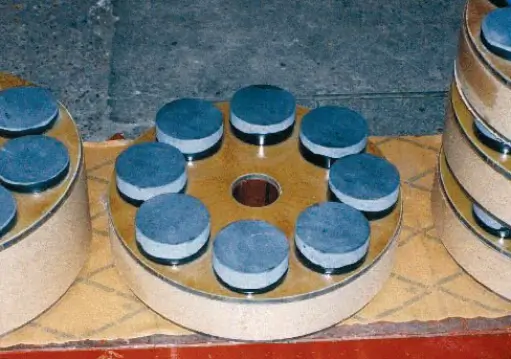
Air Gaps in Gapped Core Reactors
The presence of air gaps in these reactors serves several purposes:
- They prevent large radial flux components in the coil.
- They help maintain a consistent magnetic flux across the reactor’s height.
- They allow for impedance adjustments within specified tolerances.
The magnetic forces acting across these air gaps can cause vibrations and noise, necessitating the use of strong axial pressure to maintain alignment. This pressure is usually applied using tie rods that either pass through the core’s center or are positioned outside the windings, depending on the design.
The magnetic force between the different elements of the core is given by:
$$F=\frac{B^2_{\max}\cdot S_{ag}}{2\mu_o}$$
Where $B_\mathrm{max}=$induction in air gap[Tesla]
$S_{ag}=$cross sectional area of the air gap[m’]
$\mu_o=4\pi\cdot10^{-7}$
Operational Considerations for Shunt Reactors
- The effective operation of shunt reactors involves carefully balancing the magnetic and electrical properties of the components.
- For instance, in gapped core reactors, the primary impedance is determined by the air gaps. When the core reaches saturation, the impedance increases, a characteristic that differentiates gapped core designs from air core reactors.
Single Phase and Three Phase Shunt Reactors
1. Single Phase Shunt Reactors
Single phase reactors typically feature a magnetic frame with yokes and return legs. The tank housing these reactors is usually cylindrical, with attached radiators to manage the cooling requirements.
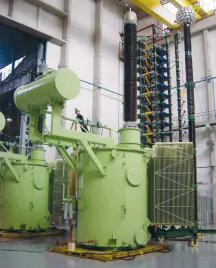
2. Three Phase Shunt Reactors
In three-phase reactors, the fluxes in each phase are displaced by 120°, resulting in zero vector sum in the yoke. This unique arrangement allows for designs both with and without return legs.
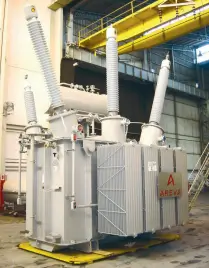
The choice between these designs depends on the customer’s impedance requirements, with reactors lacking return legs exhibiting lower zero sequence impedance due to the high reluctance in the air gap between yokes.
Cooling Mechanisms in Shunt Reactors
- The cooling system for shunt reactors varies based on their phase configuration.
- Single phase reactors might have cylindrical tanks with attached radiators.
- Three-phase reactors often feature rectangular tanks.
- Proper cooling is critical for preventing overheating and maintaining the reactor’s longevity.
Applications and Benefits of Shunt Reactors
- Shunt reactors are essential in maintaining the stability of power systems by managing reactive power, thus preventing over-voltage conditions.
- They are particularly useful in long-distance high-voltage transmission lines and extensive cable networks, where reactive power compensation is a significant concern.
Common Challenges in Shunt Reactor Operation
- Challenges include managing the noise and vibrations caused by magnetic forces in gapped core reactors and ensuring effective cooling in high voltage applications.
- Additionally, maintaining alignment and minimizing eddy current losses in the windings are critical for optimal performance.
Design Innovations in Shunt Reactors
- Recent innovations focus on enhancing the magnetic shielding of air core reactors and improving the material composition of core discs in gapped core reactors.
- Advances in insulation fluids and materials for gap spacers also contribute to better performance and reduced operational noise.
Future Trends in Shunt Reactor Technology
- Future developments are likely to include further optimization of core materials, more compact designs for high-voltage reactors, and improved cooling systems.
- The integration of digital monitoring and control systems for real-time performance assessment is another anticipated trend.
Frequently Asked Questions (FAQs)
1. What is the primary function of a shunt reactor?
Shunt reactors are used to manage reactive power and control voltage levels in power transmission systems, especially in high voltage applications.
2. What is the difference between air core and gapped core reactors?
Air core reactors lack a magnetic core and rely on the surrounding medium for flux circulation, while gapped core reactors feature a magnetic core with gaps for precise impedance control.
3. Why are air gaps important in gapped core reactors?
Air gaps help stabilize magnetic flux, maintain consistent impedance, and reduce radial flux components within the winding.
4. How does cooling work in shunt reactors?
Cooling is typically achieved using radiators attached to the reactor’s tank, with designs varying between single-phase and three-phase configurations.
5. Are shunt reactors used only in high voltage applications?
Primarily, yes. They are crucial in managing reactive power in high voltage transmission lines and extensive cable networks.
6. What are the future trends in shunt reactor technology?
Trends include advancements in core materials, compact designs, and the integration of digital monitoring systems.
Conclusion
Shunt reactors play a critical role in stabilizing power transmission systems by controlling reactive power and maintaining appropriate voltage levels. Whether through air core or gapped core designs, these devices are essential in ensuring the efficiency and reliability of modern electrical grids. As technology advances, further innovations in shunt reactor design and operation will continue to support the growing demands of global power networks.